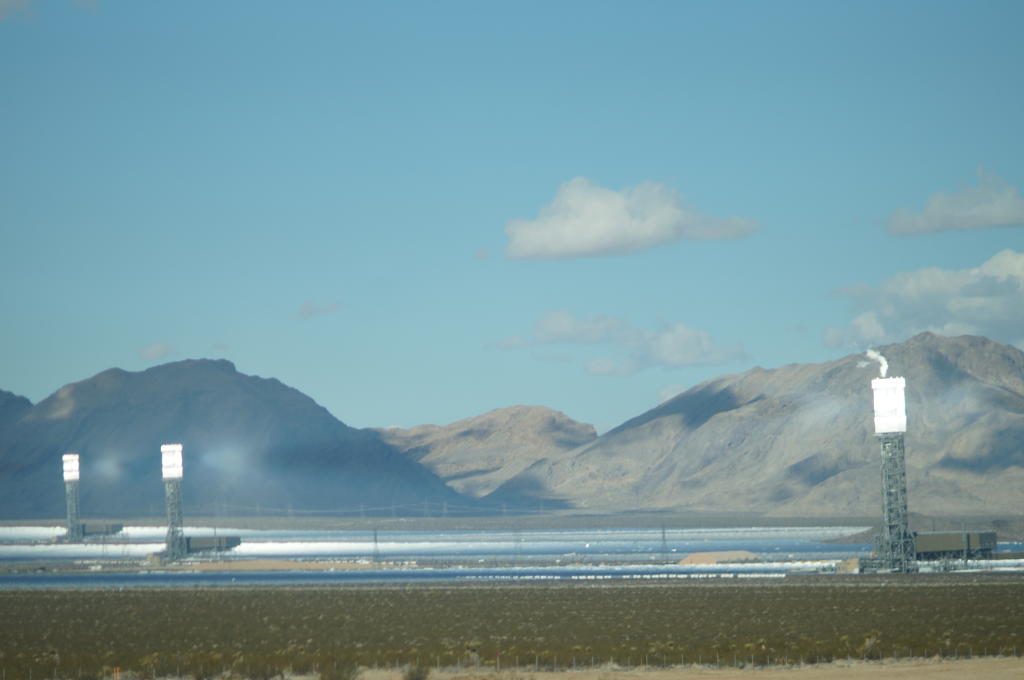
Solar thermal systems are a good example of the particle-wave dualism expressed in Planck’s constant h: E = hf. Where h is the Planck constant, f is the frequency of the light and E is the resulting energy. Thus, the higher the frequency of the light, the higher the amount of energy. Solar thermal metal collectors transform the energy of high-frequency light by generating them to an abundance of low-frequencies through Compton shifts. Glass or ceramic coatings with high visible and UV transmittance absorb the low frequency light generated by the metal because they effectively absorb infrared light (so-called heat blockers). The efficiency of the solar thermal system improves significantly with increasing size, which is also the biggest advantage of such systems compared to photovoltaic generators. One disadvantage, however, is the downstream transformation of heat into electricity with the help of heat exchangers and turbines − a problem not only in solar thermal systems.
To provide the hot gas (supercritical CO2) to the turbines, heat exchangers are necessary. These heat exchangers transfer the heat energy generated by a power plant to the working fluid in a heat engine (usually a steam turbine) that converts the heat into mechanical energy. Then, the mechanical energy is used to generate electricity. These heat exchangers are operated at ~800 Kelvin and could be more efficient if the temperature were at >1,000 Kelvin. The entire process of converting heat into electricity is called a power cycle and is a critical process in power generation by solar thermal plants. Obviously, heat exchangers are pivotal elements in this process.
Ceramics are a great material material for heat exchanger because they can withstand extreme temperature fluctuations. However, unlike metals, ceramics are not easy to shape. Relatively coarse shapes, in turn, are made quickly and easily. In contrast, metals can be easily formed and have a high mechanical strength. Metals and ceramics have been valued for centuries for their distinctive properties. For example, bronze and iron have good impact resistance and are so malleable that they have been made into complex shapes such as weapons and locks. Ceramics, like those used to make pottery, have been formed into simpler shapes. Their resistance to heat and corrosion made ceramics a valued material. A new composite of metal and ceramic (a so-called cermet) combines these properties in amazing ways. A research group led by Mario Caccia reported now in the prestigious journal Nature about a cermet with properties that makes it usable for heat exchangers in solar thermal systems.
The history of such composites goes back to the middle of the 20th century. The advent of jet engines has created a need for materials with high resistance to heat and oxidation. On top of that, they had to deal with rapid temperature changes. Their excellent mechanical strength, which often surpassed that of existing metals, was highly appreciated by the newly created aerospace industry. Not surprisingly, the US Air Force funded more research into the production of cermets. Cermets have since been developed for multiple applications, but in most cases have been used for small parts or surfaces. The newly released composite withstands extreme temperatures, high pressures and rapid temperature changes. It could increase the efficiency of heat exchangers in solar thermal systems by 20%.
To produce the composite, the authors first produced a precursor, which was subject to further processing, comparable to potting the unfired version of a clay pot. The authors compacted tungsten carbide powder into the approximate shape of the desired article (the heat exchanger) and heated it at 1,400 °C for 2 minutes to bond the parts together. They then further processed this porous preform to produce the desired final shape.
Next, the authors heated the preform in a chemically reducing atmosphere (a mixture of 4% hydrogen in argon) at 1,100 °C. At the same temperature, they immersed the preform in a tank of liquid zirconium and copper (Zr2Cu). Finally, the preform was removed by heating to 1,350 °C. In this process, the zirconium displaces the tungsten from the tungsten carbide, producing zirconium carbide (ZrC) as well as tungsten and copper. The liquid copper is displaced from the ZrC matrix as the material solidifies. The final object consists of ~58% ZrC ceramic and ~36% tungsten metal with small amounts of tungsten carbide and copper. The beauty of the method is that the porous preform is converted into a non-porous ZrC / tungsten composite of the same dimensions. The total volume change is about 1-2%.
The elegant manufacturing process is complemented by the robustness of the final product. At 800 °C, the ZrC / tungsten cermet conducts heat 2 to 3 times better than nickel based iron alloys. Such alloys are currently used in high-temperature heat exchangers. In addition to the improved thermal conductivity, the mechanical strength of the ZrC / tungsten composite is also higher than that of nickel alloys. The mechanical properties are not affected by temperatures of up to 800 ° C, even if the material has previously been subjected to heating, e.g. for cooling cycles between room temperature and 800 °C. In contrast, iron alloys, e.g. stainless steels, and nickel alloys loose at least 80% of their strength.
(Photo: Wikipedia)
This post is also available in Deutsch.