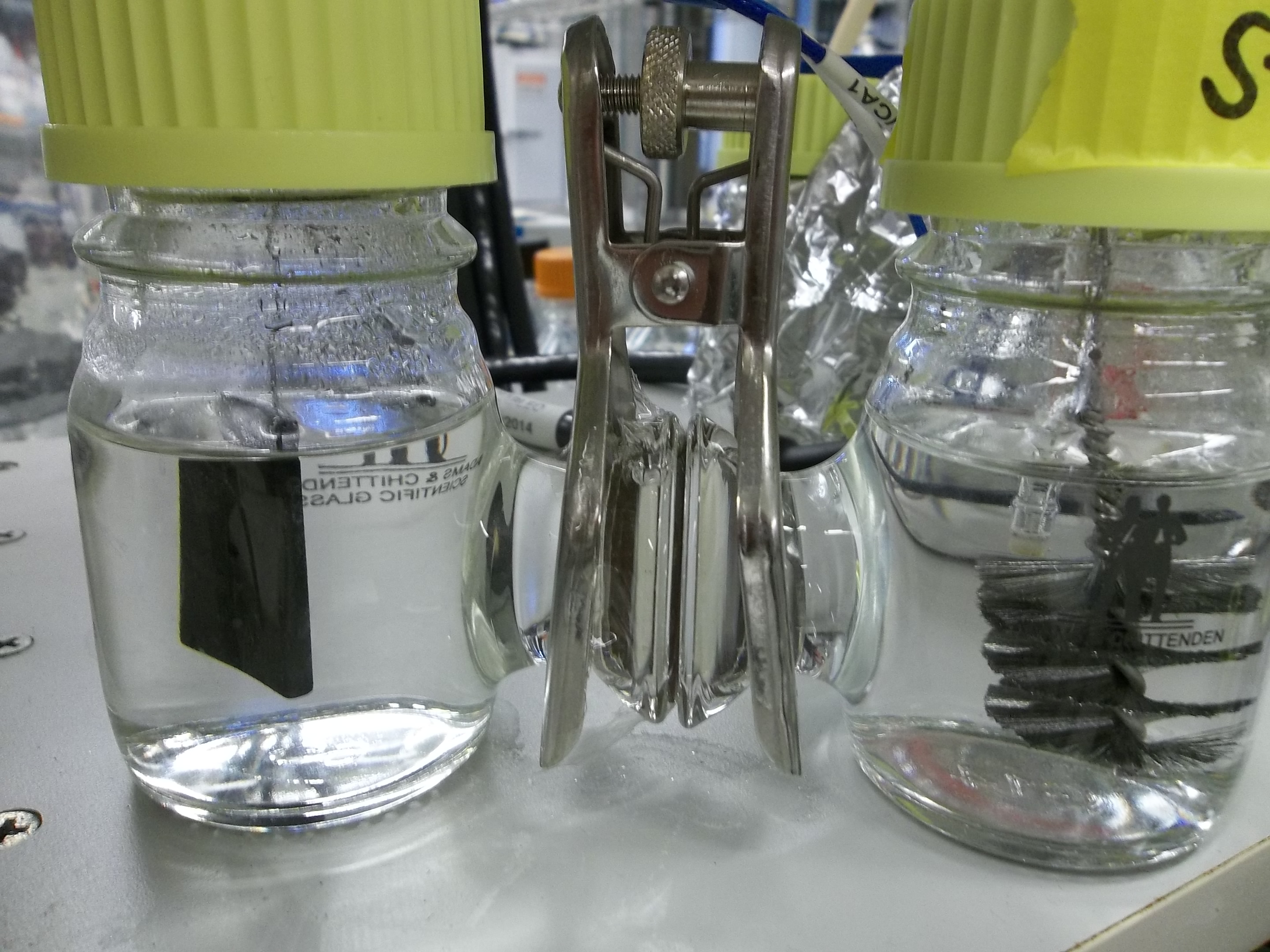
Per- and polyfluoroalkyl substances (PFAS) have been manufactured for various applications for many decades. These include medical applications, such as implants and catheters, or consumer products for firefighting, plastics, cookware, and cosmetics. Likewise, PFAS are required in countless industrial applications, such as in the automotive industry, the chemical industry and the energy sector, including hydrogen electrolysis and fuel cells (e.g. Nafion™). They help apparatuses to function properly, reduce wear and the risk of accidents. The widespread use of PFAS has led to traces of these substances entering the environment worldwide. Typical sites with higher environmental PFAS concentrations include airports, chemical plants, fire brigades, military facilities etc.
The long-term health effects of these substances are currently a matter of controversy, particularly with regard to their chemical stability (a desired property).
In addition to completely avoiding their entry into the environment, PFAS can also be eliminated from it. For example, activated charcoal is often used to adsorb PFAs onto it. However, this method is not efficient in soils. Ideally, the activated carbon itself would have to be further processed in order to reuse PFAS. This process is very energy intensive.
As for many treatment processes, microbes can be used also for PFAS. Such biological methods are called bioremediation. However, the carbon-fluorine (C-F) bonds in PFAS are among the strongest covalent bonds in organic chemistry. In addition, there are very few naturally occurring C-F bonds in nature. They only occur in small concentrations. A prominent example is fluoroacetic acid, a highly toxic compound produced by the South African poisonous gifblaar. Few microorganisms with the ability to break the C-F bond have been identified. Thus, bioremediation of PFAS is possible but a slow process.
As already described in our previous articles, bio-electrical systems can accelerate microbial conversion processes. With bio-electrical systems it is possible to offer microbes a greater electrochemical potential gradient. Since this leads to larger energy gain in microbial metabolism, such metabolic rates can be accelerated. This process is successfully employed to clean industrial waste water.
In bio-electrical systems, microorganisms along with contaminants are placed in an electrochemical apparatus. The electrodes of such a system serve as electron donors or acceptors. The biodegradation is can be measured via the electric current.
Indeed, bio-electric systems have been used to degrade fluorinated alkanes. For example, the anti-inflammatory drug dexamethasone was successfully eliminated using such an apparatus. As proposed for bio-electrical liquid fuel, designer microbiomes could also be studied for PFAS. Other drug residues, such as Prozac™, should also be examined to ensure absence from the environment.
At Frontis Energy we are looking forward to new developments for PFAS removal in bio-electrical systems.