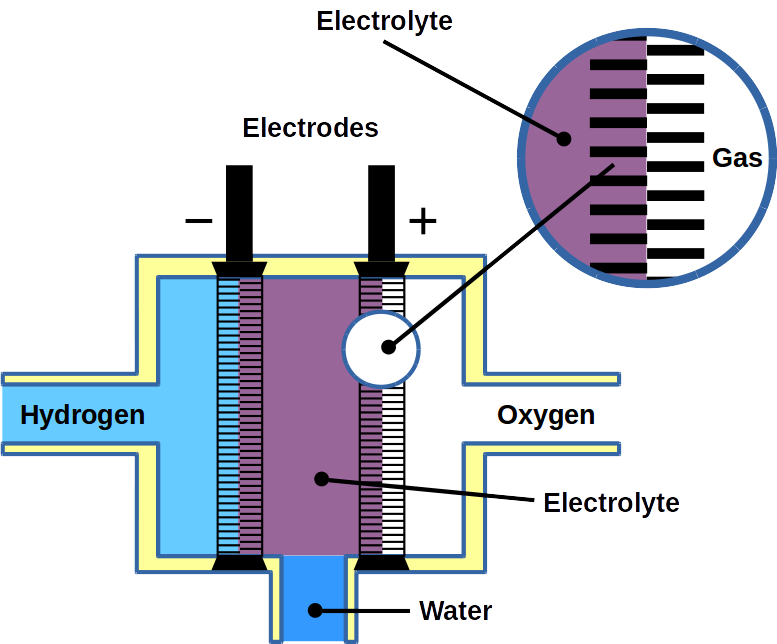
Fuel cells are a special type of galvanic cells. They can be fueled by solid, liquid, or gaseous fuel. The electrochemical oxidation of the fuel is coupled to energy gain, which is captured in form of electricity – as opposed to heat during chemical oxidation. Hence, fuel cells are direct energy converters with high efficiency. Most fuel cells achieve an energy conversion efficiency of 70-90%. If the conversion is 100%, no waste heat is produced. This ideal case of energy conversion is called ‘cold combustion’ which has been demonstrated for the first time in 1955 by Justi & Winsel. The fuel for this process is hydrogen gas, H2. It enters a porous nickel tube (gas diffusion electrode) where it is dissociated into protons and electrons according to:
H2 → 2 H+ + 2 e−
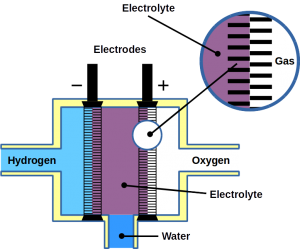
During desorption, each H atom releases a proton (H+) and an electron (e−). The electron is discharged onto the electrode, called anode, and the proton into the electrolyte. As a result of the dissociation process, the anode becomes negatively charged. On the second electrode, called cathode, oxygen gas, O2, is then charged with the electron and converted into O2− ions. The cathode becomes positively charged. Both electrodes are submerged in electrolytes which is in most cases a potassium hydroxide, KOH, solution of water. In the electrolyte, cations (H+) and anions (O2−) form water by chemical fusion. Theoretically, the efficiency is 92% accompanied by little waste heat – as opposed to normal combustion where heat of ~3,000ºC is produced.
2 H2 + O2 → H2O
Unlike heat power generators, fuel cells achieve high direct energy conversion efficiency because they avoid the additional step of heat generation. Besides shortcutting heat generation, fuel cells operate without mechanical parts and emit no noise, flue gas, or radioactivity, which puts them in focus of future developments. Due to their high energy efficiency and the high energy density of hydrogen, fuel cells are ideal for electric vehicles. In space flight, fuel cells were first used during Apollo Program between 1968 and 1972, in the Skylab Project 1973, the Apollo-Soyus Program, the Space Shuttle Program, and on board the International Space Station. There, they provide the electrical power for tools and water treatment. One benefit is that the final product of cold combustion in fuel cells is that water is the final product which is used by astronauts on their missions.
There are various types of fuel cells but all have in common that they consist of electrodes for fuel and O2 activation, and electrolytic conductors between these electrodes. Recent variations of fuel cells include methane fuel cells and microbial fuel cells. Due to the high activation energy of methane, methane fuel cells usually operate at high temperature using solid electrolytes. Microbial fuel cells, use microbes as anodic catalyst and organic matter in water as fuel. This makes them ideal for wastewater treatment.