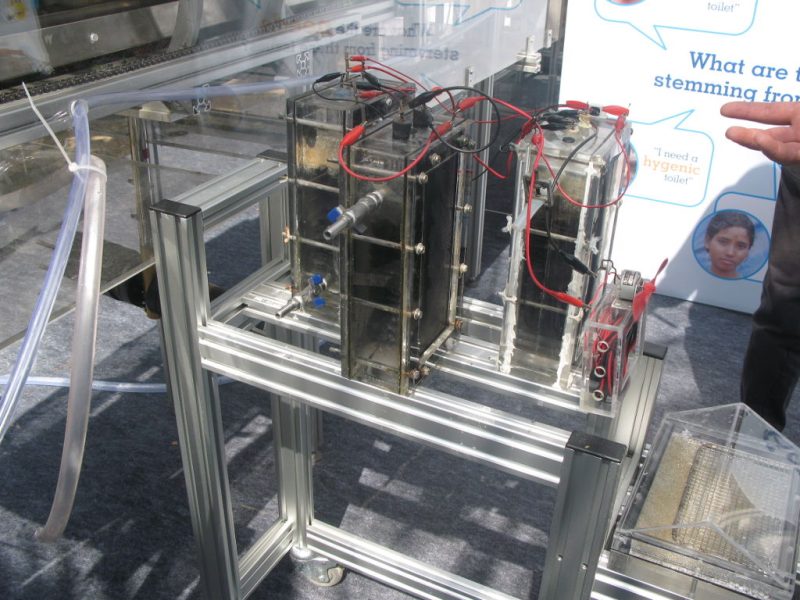
Microbial electrolysis is a technology that uses living microorganisms as electro-catalysts in electrolysis cells. The technology can be used for wastewater treatment. Earlier, we proposed that microbial electrolysis be used to decentralize wastewater treatment and biogas production. Since this is a process that converts CO2 into organic compounds using electricity it can also be used for CO2 valorization. Besides methane, such electrolysis cells produce compounds such as acetic acid (vinegar), caproic acid, and others. It is then called microbial electrosynthesis.
However, the main problem with microbial electrolysis and electrosynthesis is the long start-up time. The start-up time is the time required for the microorganisms to form a biofilm on the electrode surface and to start producing the desired products. It can range from several weeks to several months, depending on the operating conditions and the type of microorganisms. This long start-up time limits the feasibility and the scalability of microbial electrosynthesis, as well as its economic and environmental benefits.
Now, scientists of the Wageningen University in the Netherlands presented new research, which aimed to reduce the start-up time of microbial electrosynthesis. By using a novel technique that involves alternating the direction of the catholyte flow through a three-dimensional electrode they were able to reduce the startup time to only ten days. They hypothesized that this technique enhances mass transfer and biofilm formation, and thus accelerates the CO2 reduction and the product formation. This was a start-up time reduction of 50%, compared to a conventional flow-through electrode.
The alternating electrolyte flow also reduced the power consumption to 136 kWh per kg of hydrogen. After 60 days, the local hydrogen concentration at the cathode was at a maximum of 600 μM, which indicates a better mass transport and thus a more active biofilm. The researchers speculated that the alternating catholyte flow improved mass transport, because the hydrogen could be better distributed over the cathode layers. In addition, the researchers think that alternating the flow refreshed potential “dead zones” in the cathode chamber.
The pH in the catholyte was 5.8–6.8 and in optimal range for electrosynthetic microorganisms. Production of short and medium chain fatty acids was linked to the presence of microorganisms identified as Peptococcaceae and Clostridium sensu stricto 12 species. Hydrogenotrophic methanogenesis was also observed and was linked to Methanobrevibacter. The latter is a typical constituent of microbial electrolysis cells that use higher intermediate hydrogen concentrations for electrosynthesis at the cathode.
However, there are limitations of the technique, such as the energy efficiency, the product selectivity, and the scalability of microbial electrosynthesis. Such limitations are typical for bench top experiments. We are therefore looking forward to see an industrial application of this new method.