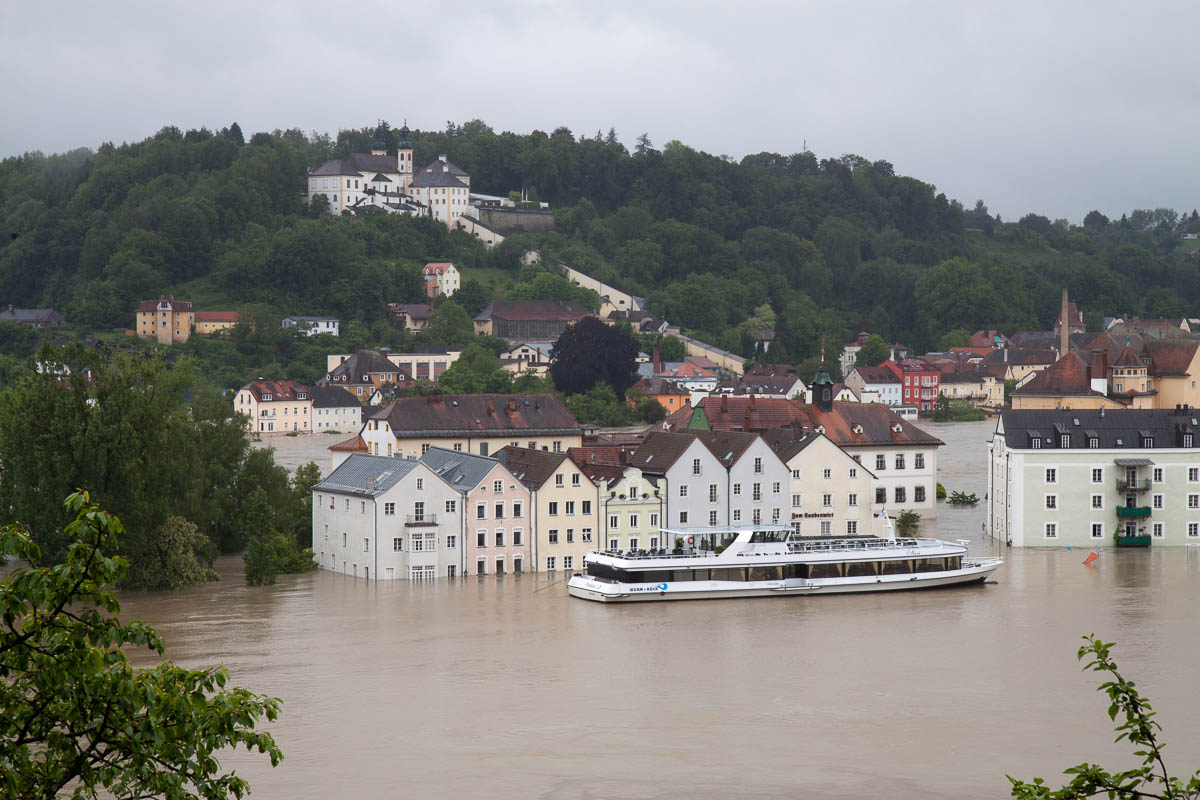
In Europe, floods are linked to high fluctuations of atmospheric pressure. These variations are also known as the North Atlantic Oscillation. Stefan Zanardo and his colleagues at Risk Management Solutions, London, UK, analyzed historical records of severe floodings in Europe since 1870. They compared patterns of atmospheric pressure at the time of the floods. When the North Atlantic Oscillation is in a positive state, a depression over Iceland drives wind and storm throughout northern Europe. In a negative state, however, it makes southern Europe moister than usual. Normally, floods occur in northern Europe. They cause the most damage if the North Atlantic Oscillation was positive in winter. If enough rain has already fallen to saturate the soil, high risk conditions for flooding are met. Air pressure in Europe may change with global warming and public administrations should take this into account when assessing flood risk in a region, the researchers say.
This is important because flooding in Europe often causes loss of life, significant property damage , and business interruptions. Global warming will further worsen this situation. Risk distribution will change as well. The frequent occurrence of catastrophic flooding in recent years has sparked strong interest in this problem in both the public and private sectors. The public sector has been working to improve early warning systems. In fact, these early warning systems have economic benefits. In addition, various risk mitigating strategies have been implemented in European countries. These include flood protection, measures to increase risk awareness, and risk transfer through better dissemination of flood insurance. The fight against the root cause, global warming that is, however, is still far behind to what is needed.
Correlations between large-scale climate patterns, and in particular the North Atlantic Oscillation, and extreme events in the water cycle on the European continent have long been described in the literature. With with more severe and more often flooding as well as alarming global warming scenarios, raising concerns over future flood-related economic losses have become the focus of public attention. Although it is known that climatic patterns also control meteorological events, it is not always clear whether this link will affect the frequency and severeness fo flooding and the associated economic losses. In their study, the researchers relate the North Atlantic Oscillation to economic flood losses.
The researchers used recent data from flood databases as well as disaster models to establish this relation. The models allowed the quantification of the economic losses that ultimately caused by the North Atlantic Oscillation. These losses vary widely between the countries within the influence of the North Atlantic Oscillation. The study shows that the North Atlantic Oscillation can well predict the average losses in the long term. Based on the predictability of the North Atlantic Oscillation, the researchers argue that, in particular, the temporal variations of the flood risks caused by climate oscillations can be forecast. This can help to take encounter catastrophic flood events early on. As a result, flood damage can be minimized or even avoided. As scientists improve their predictions for the North Atlantic Oscillation, society will be better prepared for future flooding.
(Photo: Wikipedia, Stefan Penninger, Sweden)